Shapeshifting fibers: capturing the transformation of a rogue protein
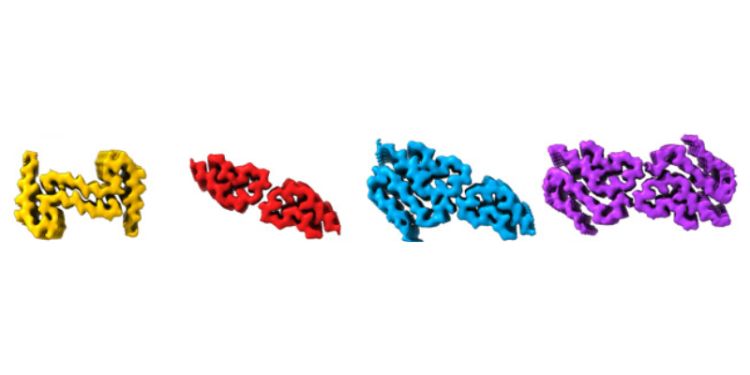
For the first time, an interdisciplinary team of researchers has revealed intricate 3D detail of how the structure of a malfunctioning protein, hIAPP, evolves over time.
The paper, Structural Evolution of Fibril Polymorphs During Amyloid Assembly, which was led by researchers, Dr Martin Wilkinson and Professors Neil Ranson and Sheena Radford at the University of Leeds, and published in Cell, describes the structures of amyloid fibrils, a type of protein build-up which accumulates in diseases such as type 2 diabetes and Alzheimer’s disease.
Scientists used Cryo-Electron Microscopy (cryoEM) – a high resolution imaging technique - to determine the structures of the hIAPP amyloid fibrils present at three distinct points of growth; an early stage when the first fibrils had formed, an intermediate point where fibrils grow dramatically, and a late time point when the fibrils had reached an apparent steady state.
Remarkably, different fibril polymorphs (various structures of the same protein sequence) were observed at different stages of assembly, with some structures only present at certain times.
In total, seven distinct hIAPP fibril polymorphs were observed as part of the study including five new structures that had not been observed previously at a single reaction time point.
Professor Neil Ranson, Professor of Structural Molecular Biology and Director of the Astbury Centre, said:
“For 30 years, scientists have been trying to understand what the end-point structure of amyloid formation looks like for proteins such as hIAPP, and thanks to powerful microscopes and data processing systems, enormous progress has been made in the last few years.
“In this study, we’ve established the new principle that amyloid structures can in fact change over time.
This new insight offers the tantalising possibility that many more undiscovered amyloid fibril structures could form during the disease process with some potentially more toxic than others. If proven, this could provide a wealth of opportunities for new treatments for diseases associated with amyloids.
The role of hIAPP
In healthy people, hIAPP is secreted from the pancreas alongside the hormone insulin, as part of our normal response to increased blood sugar. It helps to regulate blood glucose levels and the amount of food in the stomach.
When hIAPP malfunctions, also known as misfolding, it forms clumps of a protein-like substance called amyloid fibrils that are present in most people with type 2 diabetes.
But why that happens and whether it causes type 2 diabetes, or whether the amyloid forms as a result of disease, remains a mystery.
Scientists hope that by understanding the molecular events and mechanisms that take place during amyloid development, new understanding of amyloid disease and new target points for medicine will be revealed.
Demystifying amyloid transformation with cryoEM
CryoEM is a powerful imaging technique that can image cells and molecules in microscopic detail, faster than ever before.
The Titan Krios electron microscope used for this type of study, which is based in the Astbury Centre, can capture the structures of the tiny proteins and molecular machines that make our cells work.
To put this into perspective, if a typical protein were the size of a football, an actual football would be bigger than the moon.
Using this technology, scientists were able to record thousands of 2D images of the fibrils at 3 weeks, 6 weeks and 22 weeks after fibril growth was started.
Each large dataset then had to be processed to separate and group different fibrils and finally reconstruct 3D structures for each different type from which detailed atomic information for the protein could be seen.
Dr Martin Wilkinson, researcher in the School of Molecular and Cellular Biology and the first author of the study, commented:
“All high-resolution cryoEM structures of amyloid fibrils reported to date have described fibril structures at a single time-point, either taken late in disease progression, or in post-mortem samples.
What we’ve done differently is demonstrate that time is a crucial factor in amyloid formation, and hence possibly also in the progression of amyloid disease.
Professor Sheena Radford, Professor of Biophysics in the School of Molecular and Cellular Biology and the co-lead of the project added:
“Our study focuses on fibril growth in the laboratory. The next key steps will be to use the same approaches to see if fibril structure changes during time in people susceptible to amyloid disease.
If so, the structural insights we have made could transform our ability to detect and diagnose amyloid disease, to enable better and personalised medicine in the future.
“That is a long way off, but the future looks bright that thanks to the kind of methods we are developing, molecularly-directed therapeutic approaches for amyloid disease may at last be on the horizon.”
The work was funded by the Medical Research Council (MRC), the Royal Society and Wellcome.